Poster
Hepa 1-6: A murine model of hepatocellular carcinoma
In the Western population, hepatitis C, alcoholic cirrhosis, and non-alcoholic steatohepatitis (NASH) are the main underlying causes. While early stage HCC treatment options include surgical excision, liver transplantation, chemo/radio embolization and radiofrequency ablation, the standard of care for advanced HCC is targeted therapies (sorafenib and regorafenib), radiation and chemotherapy (doxorubicin, 5-FU, and cisplatin).[3] Many clinical trials are underway for unresectable or advanced stage HCC with mono or combination immunotherapy, monoclonal antibodies or oncolytic virus therapy.[1-4] These therapies have demonstrated tumor shrinkage and improved survival but are not curative with the treatment outcome contingent on pre-existing conditions such as hepatitis, liver cirrhosis, or nonalcoholic fatty liver disease (NAFLD). Hence, there is an ever-increasing need for better treatment options. To evaluate novel therapies in a preclinical platform, Labcorp has established the syngeneic HCC model Hepa 1-6.
Hepa 1-6 is a murine hepatoma derived from the BW7756 hepatoma tumor that arose spontaneously in C57L/J mice, which contrasts with most available hepatoma models (BNL, A.7R.1, MH-129, MH134 and MH-22A) that are chemically transformed or induced lines. The Hepa 1-6 tumor model established in immunocompetent mice represents a clinically relevant model for preclinical testing of immunotherapy.
In this model spotlight we present in vivo data on Hepa 1-6 tumor model growth kinetics; along with response to checkpoint inhibitors alone or in combination with focal radiation. We tested the model in its parental C57L/J strain (limited availability at The Jackson Laboratories, Bar Harbor, ME) and the more readily available, histocompatable C57BL/6 strain.
Hepa 1-6 Growth Parameters
Growth kinetics following subcutaneous implant show that the model successfully establishes in both mouse strains (Fig. 1). The tumors have a median doubling time of ~5-6 days with no adverse body weight changes (data not shown). This steady growth rate allows for a three-week therapeutic window to evaluate anti-tumor responses.
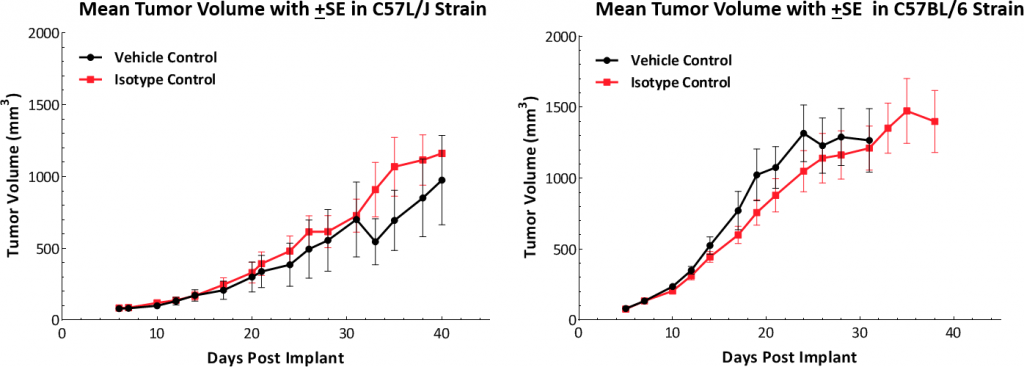
Fig. 1: Hepa 1-6 tumor growth kinetics in C57L/J and C57BL/6 mice.
Hepa 1-6 Tumor Immune Profile
To understand the immune profile of the Hepa 1-6 tumors, baseline immune composition was analyzed by flow cytometry and is shown in Fig. 2. The immune profile was established from five untreated Hepa 1-6 tumors grown in the C57L/J strain to ~750-1000mm3 in volume. Tumors have a relatively high CD45+ cell population (mean ~60%) of which the T-lymphocytes (CD8+ T cells and CD4+ T helper cells) were moderately represented (mean ~11% and ~6%, respectively). The myeloid population was represented predominantly by G-MDSC (mean ~30%) followed by M-MDSC (mean ~11%). The CD8+ T cell infiltration within the tumor microenvironment is suggestive of an immunologically responsive or ?warm? tumor model.
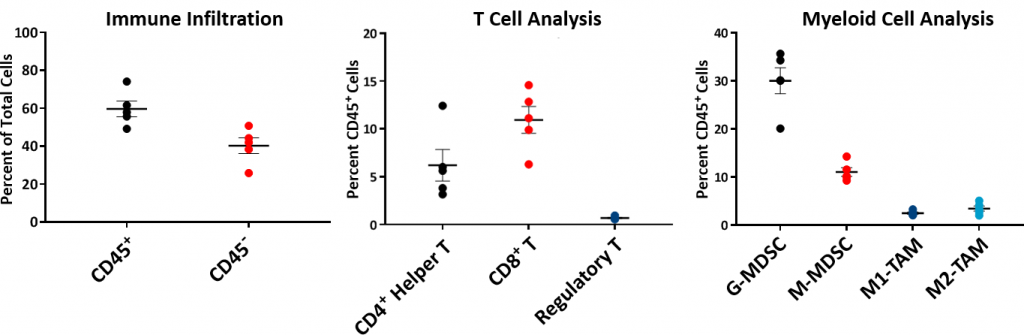
Fig. 2: Baseline Hepa 1-6 Tumor Immune Profile in C57L/J mice.
Hepa 1-6 Response to Checkpoint Inhibitors
Hepa 1-6 response to immunomodulatory agents was evaluated in established tumor bearing (mean tumor volume ~100mm3) C57BL/6 mice treated with checkpoint blockade antibodies (anti-mPD-1, anti-mPD-L1, and anti-mCTLA-4). The controls, vehicle (PBS) and isotype (Rat IgG2b clone LTF-2), showed comparable growth kinetics (Fig. 1). Single agent checkpoint inhibitors anti-mPD-1 and anti-mCTLA-4 elicited a strong response leading to complete tumor regression (CR) and 100% tumor free survivors (TFS). Anti-mPD-L1 treatment also produced a good response in a subset of animals resulting in CR and 5/10 TFS (Fig 3). Treatment with checkpoint inhibitor antibodies was not associated with any significant body weight changes relative to control animals (data not shown). The response to immunotherapy strongly supports the characterization of Hepa 1-6 tumors as immunologically ?hot.?
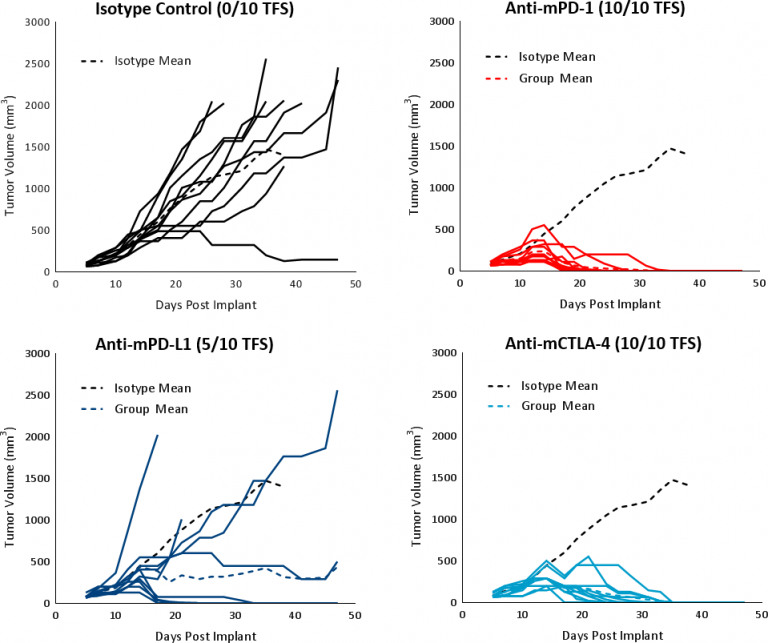
Fig. 3: Hepa 1-6 tumor response to checkpoint inhibitors in C57BL/6 mice.
Response to Focal Radiation
Clinically, patients with non-metastatic HCC receive one or more liver directed therapy (LDT) such as radiotherapy (RT) or stereotactic body radiation therapy (SBRT).[5] RT can result in tumor shrinkage and alter the tumor microenvironment to a favorable tumor suppressive milieu. To test the response of Hepa 1-6 tumors to RT, we used the Small Animal Radiation Research Platform (SARRP; Xstrahl) to deliver focal beam radiation to targeted areas. Focal radiation doses of 5 and 10Gy were tested on subcutaneous Hepa 1-6 tumors in C57BL/6 mice. Treatment showed a dose dependent response and resulted in >20 days tumor growth delay (TGD) and tumor free survivors (2/10 and 8/10, respectively) (Fig. 4). A dose of 5Gy provided a moderate monotherapy response with increased life span and is recommended for future combination studies.
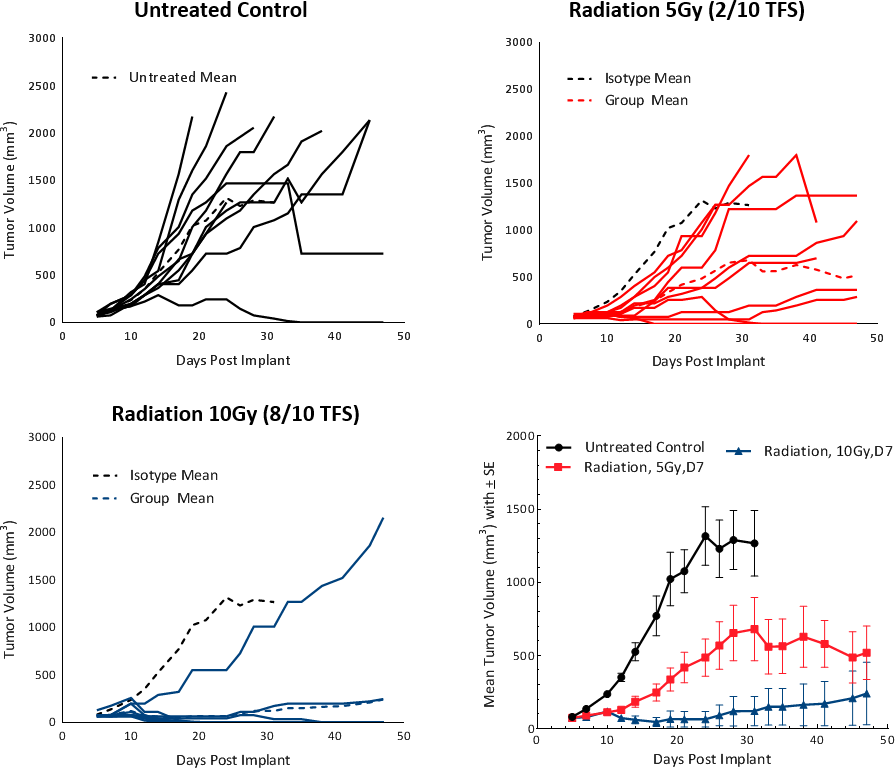
Fig. 4: Hepa 1-6 tumor response to radiation treatment in C57BL/6 mice.
Hepa 1-6 Response to Sorafenib
Sorafenib, a multi-tyrosine kinase inhibitor that blocks the activity of Raf kinase, Src, VEGF and PDGF receptors, used as first line treatment in HCC patients, was tested on Hepa 1-6 tumors. Treatment resulted in tumor growth delay of 25.4 days and extended overall survival but resulted in no TFS (Fig 5). These results are comparable to outcomes from clinical studies which showed partial response extending median overall survival from 7.9 months in the control group to 10.7 months in the treated group.[1]
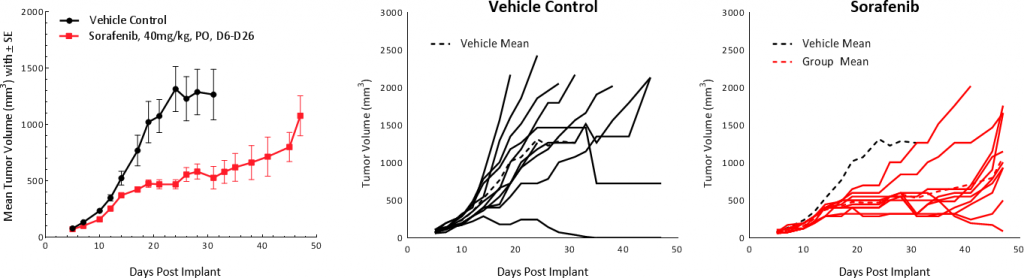
Fig. 5: Hepa 1-6 tumor response to sorafenib treatment in C57BL/6 mice.
These preliminary data suggest that the Hepa 1-6 model of HCC has a favorable immune profile and can be employed as a robust preclinical immuno-oncology model. Our data supports the use of this model in investigating immunotherapy agents as monotherapy or in combination with radiation, small molecules, standards of care, and other novel therapeutics.
Please contact us to speak with our scientists about how Hepa 1-6 can be used for your next immuno-oncology study.
References
[1]Medavaram, S and Zhang Y, 2018. Emerging therapies in advanced hepatocellular carcinoma. Exp Hematol Oncol 7:17.
[2]Llovet JM, Montal R, Sia D and Finn RS, 2018. Molecular therapies and precision medicine for hepatocellular carcinoma. Nat Rev Clin Oncol. doi: 10.1038/s41571-018-0073-4. [Epub ahead of print] PMID:30061739.
[3]Pinter M & Peck-Radosvljevic M. 2018. Review article: systemic treatment of hepatocellular carcinoma. 2018. Aliment Pharmacol Thera, Jul 23. doi: 10.1111/apt.14913. [Epub ahead of print] Review. PMID: 30039640.
[4]Waidmann O. 2018. Recent developments with immunotherapy for hepatocellular carcinoma. Expert Opin Biol Ther. Aug;18(8):905-910.